Key Takeaways
- Parkinson’s disease is a progressive neurological disorder that affects movement and coordination.
- Oxidative stress and excess iron are significant factors in the progression of Parkinson’s.
- Early detection and lifestyle changes can help manage symptoms and slow the disease.
- Current treatments focus on symptom management, with ongoing research into neuroprotective therapies.
- Support systems for patients and caregivers are crucial for maintaining quality of life.
Introduction to Parkinson’s Disease
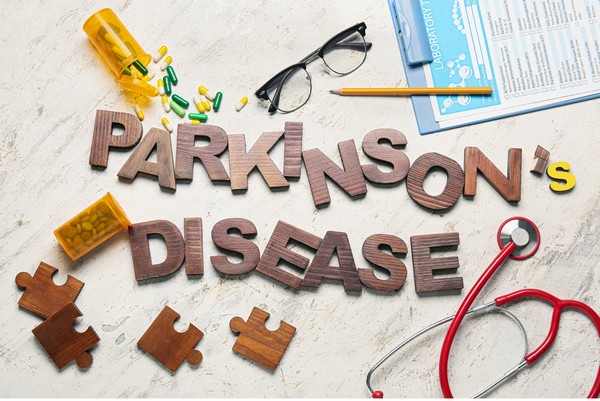
Parkinson’s disease is a neurological disorder that primarily affects movement, causing symptoms like
- Tremors
- Stiffness
- Difficulty with balance and coordination
It is the second most common neurodegenerative disorder after Alzheimer’s disease, affecting millions of people worldwide.
As the disease progresses, it leads to increasing disability, making early detection and management critical.
Causes and Risk Factors
Genetic Factors:
Parkinson’s disease has a genetic component, with certain gene mutations such as LRRK2, PARK7, and SNCA increasing the risk of developing the condition.
However, most cases are sporadic, meaning they occur in people with no known family history of the disease.
Environmental and Lifestyle Factors:
Environmental factors such as exposure to pesticides, heavy metals, and other toxins have been linked to an increased risk of Parkinson’s Disease.
One common toxin found in various plants is called lectins. Lectins are used quite often in Parkinson’s disease research. Multiple studies show lectins traveling from the gut to the brain.
A Danish study found cutting the nerve connecting the gut to the brain (vagotomy) lowers the risk of developing Parkinson’s disease.
Repeated head injuries and the use of certain medications can also contribute to the development of the disease.
Age and Gender:
Parkinson’s disease predominantly affects older adults, with the average age of onset around 60.
Men are more likely to develop Parkinson’s Disease than women, possibly due to differences in genetics and exposure to environmental risks.
The Role of Iron in Parkinson’s:
Excess iron in the brain can be a major factor in the development of Parkinson’s Disease.
Iron can accumulate in the substantia nigra, a region of the brain heavily affected by Parkinson’s, leading to oxidative stress and neuronal damage.
Oxidative Stress & Parkinson’s Disease
Oxidative Stress and Neuronal Damage:
Oxidative stress occurs when there is an imbalance between free radicals and antioxidants in the body, leading to cellular damage.
In Parkinson’s disease, oxidative stress contributes to the degeneration of dopamine-producing neurons in the substantia nigra, a key brain area involved in movement control.
Iron and the Fenton Reaction:
Excess iron in the brain catalyzes the production of reactive oxygen species (ROS) through the Fenton reaction.
These ROS cause significant damage to neurons, exacerbating the progression of Parkinson’s disease.
Importance of Mineral Balance:
Maintaining a balance of essential minerals, particularly ensuring optimal levels of bioavailable copper and magnesium, is important in mitigating oxidative stress.
These minerals help protect against the harmful effects of excess iron and support overall brain health.
Inflammation and Iron Accumulation:
Chronic inflammation can lead to increased iron accumulation in the brain, worsening oxidative stress and contributing to the severity of Parkinson’s symptoms.
Addressing inflammation is an important aspect of managing the disease.
Brain Changes in Parkinson’s Disease
Loss of Dopamine Neurons:
Parkinson’s disease leads to the progressive loss of dopamine-producing neurons in the substantia nigra.
Dopamine is a neurotransmitter essential for regulating movement, and its depletion results in the motor symptoms characteristic of Parkinson’s.
Lewy Bodies and Alpha-Synuclein:
Lewy bodies, abnormal clumps of protein made of alpha-synuclein, are found in the brains of people with Parkinson’s.
These deposits disrupt normal brain function and are thought to play a key role in the progression of the disease.
Neurotransmitter Imbalances:
Oxidative stress and mineral imbalances, particularly involving iron and copper, disrupt neurotransmitter systems in the brain.
The decline in dopamine levels directly impacts motor control, while other neurotransmitters affected by the disease contribute to non-motor symptoms such as mood changes and cognitive decline.
Diagnosis of Parkinson’s Disease
Early Signs and When to See a Doctor:
Early symptoms of Parkinson’s include tremors, stiffness, slowness of movement (bradykinesia), and difficulties with balance.
If you or a loved one notices these symptoms, it’s important to consult a healthcare professional for an evaluation.
Diagnostic Tools and Tests:
Parkinson’s is primarily diagnosed through clinical evaluation, including a review of symptoms and physical examination.
Imaging techniques like MRI and PET scans can support the diagnosis, although there are no definitive tests for Parkinson’s.
Biomarkers, such as those indicating oxidative stress or iron levels, are an area of active research.
Importance of Early Diagnosis:
Early diagnosis allows for better management of symptoms and planning for future care. It also opens the door to participate in clinical trials for new treatments, which may slow disease progression.
Treatments and Management Strategies
Medications for Symptom Management:
Several medications are available to help manage Parkinson’s symptoms. The most common treatment is levodopa, which replenishes dopamine levels in the brain.
Other medications include dopamine agonists, which mimic dopamine’s effects, and MAO-B inhibitors, which prevent the breakdown of dopamine.
Addressing Oxidative Stress and Iron Regulation:
Managing oxidative stress is helpful in Parkinson’s treatment. Supplements like colostrum, which contains lactoferrin, can help regulate iron levels and reduce oxidative stress.
Ensuring optimal levels of bioavailable copper and magnesium is also important for protecting brain health.
Non-Pharmacological Interventions:
In addition to medications, physical therapy, exercise, and other lifestyle changes play a significant role in managing Parkinson’s symptoms.
Regular exercise can improve mobility, balance, and overall well-being, while physical therapy can help with specific movement challenges.
Emerging Therapies and Research:
Research into Parkinson’s is ongoing, with promising developments in neuroprotective treatments, gene therapy, and new drugs targeting the underlying causes of the disease.
These emerging therapies offer hope for slowing the progression of Parkinson’s and improving the quality of life for those affected.
Lifestyle and Prevention
Diet and Nutrition:
A diet rich in whole, nutrient-dense foods, close to ancestral diets, can help manage and potentially prevent Parkinson’s Disease.
It’s important to avoid ultra-processed foods, excess carbohydrates, and foods artificially fortified with iron.
Eat more foods that support optimal mineral balance, such as grass-fed meat, eggs and seafood.
Physical Activity:
Regular exercise is essential for maintaining mobility, balance, and overall brain health in people with Parkinson’s.
Physical activity has been shown to slow the progression of the disease and improve the quality of life.
Cognitive Engagement:
Mental stimulation through activities like puzzles, reading, and social interaction is important for maintaining cognitive function.
Keeping the mind active can help delay the onset of cognitive decline associated with Parkinson’s.
Sleep and Stress Management:
Quality sleep and stress reduction are vital for managing Parkinson’s symptoms.
Poor sleep and chronic stress can exacerbate symptoms, so practicing good sleep hygiene and stress management techniques, such as mindfulness and meditation, is important.
Frequently Asked Questions (FAQ)
What are the early signs of Parkinson’s?
Early signs include tremors, stiffness, slowness of movement, and balance issues.
Can Parkinson’s be prevented?
While there is no guaranteed way to prevent Parkinson’s, maintaining a healthy lifestyle with a balanced diet, regular exercise, and mental stimulation can reduce the risk.
How does oxidative stress contribute to Parkinson’s?
Oxidative stress damages brain cells by producing harmful free radicals, which can accelerate the progression of Parkinson’s.
What role does iron play in the development of Parkinson’s?
Excess iron can contribute to oxidative stress, leading to neuronal damage and increasing the risk of Parkinson’s.
Regulating iron levels with optimized bioavailable copper, and ensuring a balance of essential minerals with support like lactoferrin from colostrum can help mitigate this risk.
How can diet and lifestyle changes help prevent or manage Parkinson’s?
A diet rich in whole, nutrient-dense foods, regular physical activity, mental engagement, and good sleep hygiene can help prevent or manage Parkinson’s by supporting brain health and
Research
Anselmi, L., Bove, C., Coleman, F. H., Le, K., Subramanian, M. P., Venkiteswaran, K., Subramanian, T., & Travagli, R. A. (2018). Ingestion of subthreshold doses of environmental toxins induces ascending Parkinsonism in the rat. Npj Parkinson's Disease, 4(1), 1-10. https://doi.org/10.1038/s41531-018-0066-0
Batey RG, Lai Chung Fong P, Shamir S, Sherlock S. A non-transferrin-bound serum iron in idiopathic hemochromatosis. Dig Dis Sci. 1980 May;25(5):340-6. doi: 10.1007/BF01308057. PMID: 7371472.
Boddaert, N., Le Quan Sang, K. H., Rötig, A., Leroy-Willig, A., Gallet, S., Brunelle, F., Sidi, D., Thalabard, J., Munnich, A., & Cabantchik, Z. I. (2007). Selective iron chelation in Friedreich ataxia: Biologic and clinical implications. Blood, 110(1), 401-408. https://doi.org/10.1182/blood-2006-12-065433
DiNicolantonio, J.J., Mangan, D. and O’Keefe, J.H., 2018. The fructose–copper connection: Added sugars induce fatty liver and insulin resistance via copper deficiency. Journal of Metabolic Health, [online] 3(1).
https://doi.org/10.4102/jir.v3i1.43.
Duyn, J.H., 2010. Study of brain anatomy with high-field MRI: recent progress. Magnetic Resonance Imaging, [online] 28(8), pp.1210–1215. https://doi.org/10.1016/j.mri.2010.02.007.
Fillebeen, C., Descamps, L., Dehouck, M.-P., Fenart, L., Benaïssa, M., Spik, G., Cecchelli, R. and Pierce, A., 1999. Receptor-mediated Transcytosis of Lactoferrin through the Blood-Brain Barrier. Journal of Biological Chemistry, [online] 274(11), pp.7011–7017. https://doi.org/10.1074/jbc.274.11.7011.
Galaris, D., Barbouti, A. and Pantopoulos, K., 2019. Iron homeostasis and oxidative stress: An intimate relationship. Biochimica et Biophysica Acta (BBA) - Molecular Cell Research, [online] 1866(12), p.118535. https://doi.org/10.1016/j.bbamcr.2019.118535.
Greenberg, G.R. and Wintrobe, M.M., 1946. A LABILE IRON POOL. Journal of Biological Chemistry, [online] 165(1), pp.397–398. https://doi.org/10.1016/s0021-9258(17)41250-6.
Gröger, A. and Berg, D., 2012. Does structural neuroimaging reveal a disturbance of iron metabolism in Parkinson’s disease? Implications from MRI and TCS studies. Journal of Neural Transmission, [online] 119(12), pp.1523–1528. https://doi.org/10.1007/s00702-012-0873-0.
Gutteridge, J.M.C. and Halliwell, B., 2018. Mini-Review: Oxidative stress, redox stress or redox success? Biochemical and Biophysical Research Communications, [online] 502(2), pp.183–186.
https://doi.org/10.1016/j.bbrc.2018.05.045.
Hentze, M.W., Muckenthaler, M.U., Galy, B. and Camaschella, C., 2010. Two to Tango: Regulation of Mammalian Iron Metabolism. Cell, [online] 142(1), pp.24–38. https://doi.org/10.1016/j.cell.2010.06.028.
Ke, Y. and Qian, Z.M., 2007. Brain iron metabolism: Neurobiology and neurochemistry. Progress in Neurobiology, [online] 83(3), pp.149–173. https://doi.org/10.1016/j.pneurobio.2007.07.009.
Kenkhuis, B., Bush, A.I. and Ayton, S., 2023. How iron can drive neurodegeneration. Trends in Neurosciences, [online] 46(5), pp.333–335.
https://doi.org/10.1016/j.tins.2023.02.003.
Kruszewski, M., 2003. Labile iron pool: the main determinant of cellular response to oxidative stress. Mutation Research/Fundamental and Molecular Mechanisms of Mutagenesis, [online] 531(1–2), pp.81–92.
https://doi.org/10.1016/j.mrfmmm.2003.08.004.
Liddle, R.A., 2018. Parkinson’s disease from the gut. Brain Research, [online] 1693, pp.201–206. https://doi.org/10.1016/j.brainres.2018.01.010.
Liu, B., Fang, F., Pedersen, N.L., Tillander, A., Ludvigsson, J.F., Ekbom, A., Svenningsson, P., Chen, H. and Wirdefeldt, K., 2017. Vagotomy and Parkinson disease. Neurology, [online] 88(21), pp.1996–2002. https://doi.org/10.1212/wnl.0000000000003961.
Mason, S. T., & Fibiger, H. C. (1979). Regional topography within noradrenergic locus coeruleus as revealed by retrograde transport of horseradish peroxidase. Journal of Comparative Neurology, 187(4), 703-724. https://doi.org/10.1002/cne.901870405
Moin, K., Funk, C., Josephs, M., Coombes, K., Yeakle, M., Gala, D., & Ahmed-Khan, M. (2022). Gut-brain axis: Review on the association between Parkinson’s disease and plant lectins. Archive of Clinical Cases, 9(4), 177-183. https://doi.org/10.22551/2022.37.0904.10228
Moos, T., Nielsen, T.R., Skjørringe, T. and Morgan, E.H., 2007. Iron trafficking inside the brain. Journal of Neurochemistry, [online] 103(5), pp.1730–1740. https://doi.org/10.1111/j.1471-4159.2007.04976.x.
Moos T, Morgan EH. Transferrin and transferrin receptor function in brain barrier systems. Cell Mol Neurobiol. 2000 Feb;20(1):77-95. doi: 10.1023/a:1006948027674. PMID: 10690503.
Muckenthaler MU, Galy B, Hentze MW. Systemic iron homeostasis and the iron-responsive element/iron-regulatory protein (IRE/IRP) regulatory network. Annu Rev Nutr. 2008;28:197-213. doi: 10.1146/annurev.nutr.28.061807.155521. PMID: 18489257.
Petok, J.R., Merenstein, J.L. and Bennett, I.J., 2024. Iron content affects age group differences in associative learning-related fMRI activity. NeuroImage, [online] 285, p.120478. https://doi.org/10.1016/j.neuroimage.2023.120478.
Rouault, T. A. (2013). Iron metabolism in the CNS: Implications for neurodegenerative diseases. Nature Reviews Neuroscience, 14(8), 551-564. https://doi.org/10.1038/nrn3453
Shan, L., Heusinkveld, H.J., Paul, K.C., Hughes, S., Darweesh, S.K.L., Bloem, B.R. and Homberg, J.R., 2023. Towards improved screening of toxins for Parkinson’s risk. npj Parkinson’s Disease, [online] 9(1). https://doi.org/10.1038/s41531-023-00615-9.
Sian‐Hülsmann, J., Mandel, S., Youdim, M.B.H. and Riederer, P., 2011. The relevance of iron in the pathogenesis of Parkinson’s disease. Journal of Neurochemistry, [online] 118(6), pp.939–957. https://doi.org/10.1111/j.1471-4159.2010.07132.x.
Wallander, M.L., Leibold, E.A. and Eisenstein, R.S., 2006. Molecular control of vertebrate iron homeostasis by iron regulatory proteins. Biochimica et Biophysica Acta (BBA) - Molecular Cell Research, [online] 1763(7), pp.668–689. https://doi.org/10.1016/j.bbamcr.2006.05.004.
Weinreb, O., Amit, T., Mandel, S., Kupershmidt, L. and Youdim, M.B.H., 2010. Neuroprotective Multifunctional Iron Chelators: From Redox-Sensitive Process to Novel Therapeutic Opportunities. Antioxidants & Redox Signaling, [online] 13(6), pp.919–949. https://doi.org/10.1089/ars.2009.2929.
Wang, J. and Pantopoulos, K., 2011. Regulation of cellular iron metabolism. Biochemical Journal, [online] 434(3), pp.365–381. https://doi.org/10.1042/bj20101825.
Ward, R.J., Zucca, F.A., Duyn, J.H., Crichton, R.R. and Zecca, L., 2014. The role of iron in brain ageing and neurodegenerative disorders. The Lancet Neurology, [online] 13(10), pp.1045–1060.
https://doi.org/10.1016/s1474-4422(14)70117-6.
Zecca, L., Gallorini, M., Schünemann, V., Trautwein, A. X., Gerlach, M., Riederer, P., Vezzoni, P., & Tampellini, D. (2001). Iron, neuromelanin and ferritin content in the substantia nigra of normal subjects at different ages: Consequences for iron storage and neurodegenerative processes. Journal of Neurochemistry, 76(6), 1766-1773. https://doi.org/10.1046/j.1471-4159.2001.00186.x
Say Goodbye to Depression with Natural Remedies
Key Takeaways Regular exercise, sufficient sleep, and a diet full of bioavailable nutrients support mental health. Spending time in nature, like forest bathing and gardening,…
Alzheimer’s Disease: Symptoms, Causes, Treatment
Key Takeaways Alzheimer’s disease is a progressive neurodegenerative disorder affecting memory, thinking, and behavior. Oxidative stress, including from excess iron, plays a significant role in…
Fight, Flight, Freeze, Fawn: Stress Response Examined
Key Takeaways The stress response includes four primary reactions, each serving as a survival mechanism. The fight response involves confronting the threat, often with aggression…
Mastering Emotional Healing: Your Guide to Recovery
Key Highlights Emotional healing is a gradual process requiring patience and self-compassion. Acknowledge and accept your feelings, and identify sources of emotional pain. Embrace vulnerability,…
Supporting Mental Health with Gut Health
Key Takeaways Gut-Brain Connection: Gut health is directly linked to mental wellbeing through the gut-brain axis. Probiotics: Beneficial bacteria that help regulate mood and support…
Reduce Stress through Pet Therapy
Key Highlights Pet therapy, or animal-assisted therapy, boosts health. Interacting with animals lowers cortisol, blood pressure, and heart rate. Therapy, service, and companion animals are…
Mental Benefits of Learning a New Language
Key Takeaways: Enhances brain volume and memory. Improves cognitive flexibility, multitasking, and executive functions. Delays cognitive decline and boosts emotional intelligence. Increases empathy, cultural awareness,…
Anxiety: Causes, Symptoms, and Management
Key Takeaways: Anxiety can disrupt daily life when it becomes overwhelming. Common symptoms include restlessness, rapid heartbeat, and difficulty concentrating. Causes include stress, genetics, and…
GABA (gamma-aminobutyric acid)
Key Takeaways GABA is a neurotransmitter that helps calm the nervous system. Low GABA levels can lead to anxiety, stress, and sleep problems. Natural precursors…
Dementia: Causes, Symptoms, and Management
Key Takeaways: Dementia involves a decline in cognitive function affecting memory, thinking, and daily life. Common causes include genetic factors, aging, and certain medical conditions….
Emotional Freedom Technique (EFT): Tap to relieve stress
Key Highlights Learn about the origins and principles of Emotional Freedom Technique (EFT) for stress relief. Understand the science-backed mechanisms of how EFT works on…