Key Takeaways
- The Randle Cycle explains how the body chooses between burning glucose and fatty acids for energy.
- Enzymes and hormones play a key role in regulating the balance between glucose and fat metabolism.
- This cycle influences insulin sensitivity, which is important for maintaining healthy blood sugar levels.
- Imbalances in The Randle Cycle can contribute to metabolic issues like obesity and type 2 diabetes.
- Diet and exercise can help manage and optimize The Randle Cycle, improving overall metabolic health.
Introduction to The Randle Cycle
The Randle Cycle is a concept that describes how the body decides whether to burn glucose (sugar) or fatty acids (fats) for energy.
This process is important for understanding how our metabolism functions and how it can impact health.
Biochemical Pathway of The Randle Cycle
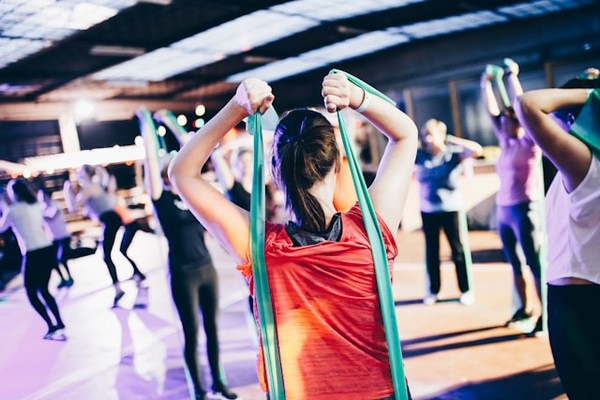
Interaction Between Glucose and Fatty Acid Metabolism
The body breaks down carbohydrates into glucose and fats into fatty acids after eating. These two fuels compete to be used for energy, with glucose usually being the preferred fuel when it is abundant.
However, when fats are more available, the body switches to burning fatty acids.
Enzymes and hormones, like insulin, help the body decide which fuel to burn, depending on the availability of these nutrients.
Role of The Randle Cycle in Different States
The Randle Cycle functions differently during fasting, after meals, and during exercise. During fasting, the body tends to burn more fats because glucose levels are lower.
After consuming a meal rich in carbohydrates, the body shifts to burning glucose.
The balance between glucose and fat burning also varies with exercise intensity and duration, with low-intensity activities favoring fat burning and high-intensity activities favoring glucose.
Implications of The Randle Cycle
The Cycle’s Role in Insulin Resistance
The Randle Cycle can affect insulin sensitivity, which is essential for regulating blood sugar levels.
The Randle Cycle and Metabolic Disorders
Disruptions in The Randle Cycle can contribute to metabolic conditions such as obesity and type 2 diabetes.
If the body consistently chooses to burn fats, it might store excess glucose as fat, leading to weight gain.
Conversely, an over-reliance on glucose burning can also contribute to fat accumulation.
Practical Applications
Nutritional Strategies to Influence The Randle Cycle
A balanced diet that includes healthy fats can help regulate The Randle Cycle.
Minimising carbohydrate intake can enhance the body’s ability to burn fat, which may benefit metabolic health.
Exercise and The Randle Cycle
Exercise plays a significant role in influencing The Randle Cycle. Low-intensity exercise, such as walking, encourages fat burning, while high-intensity exercise relies more on glucose.
Varying exercise routines can help the body become more adaptable in switching between fuel sources.
FAQs
What is the primary function of The Randle Cycle?
The Randle Cycle determines whether the body burns glucose or fatty acids for energy, ensuring a balance in fuel usage.
How can diet affect The Randle Cycle?
A balanced diet that minimizes carbohydrate intake can help regulate The Randle Cycle, improving metabolic health.
Can The Randle Cycle be modified by lifestyle changes?
Yes, diet and exercise significantly impact The Randle Cycle, allowing for better management of energy use and metabolic health.
Why is The Randle Cycle important in metabolic research?
The Randle Cycle is crucial for understanding metabolic diseases like diabetes and obesity, helping researchers develop effective treatments.
Research
Bevilacqua, S., Bonadonna, R., Buzzigoli, G., Boni, C., Ciociaro, D., Maccari, F., Giorico, M. A., & Ferrannini, E. (1987). Acute elevation of free fatty acid levels leads to hepatic insulin resistance in obese subjects. Metabolism, 36(5), 502-506. https://doi.org/10.1016/0026-0495(87)90051-5
Bevilacqua, S., Buzzigoli, G., Bonadonna, R., Brandi, L.S., Oleggini, M., Boni, C., Geloni, M. and Ferrannini, E. (1990). Operation of Randle's cycle in patients with NIDDM. Diabetes, 39(3), 383-389.
Boros, L.G., Huang, D. and Heaney, A.P. (2012). Fructose drives glucose via direct oxidation and promotes palmitate/oleate co-release from Hepg2 cells: relevance with the Randle cycle. Metabolomics, 2(107), 2153-0769.
Bonadonna, R. C., Groop, L. C., Simonson, D. C., & DeFronzo, R. A. (1994). Free fatty acid and glucose metabolism in human aging: Evidence for operation of the Randle cycle. American Journal of Physiology-Endocrinology and Metabolism. https://doi.org/10.1152/ajpendo.1994.266.3.E501
Chung, S.T., Chacko, S.K., Sunehag, A.L. and Haymond, M.W. (2015). Measurements of gluconeogenesis and glycogenolysis: a methodological review. Diabetes, 64(12), 3996-4010.
DiNicolantonio, J.J., Mangan, D. and O’Keefe, J.H., 2018. The fructose–copper connection: Added sugars induce fatty liver and insulin resistance via copper deficiency. Journal of Metabolic Health, [online] 3(1).
https://doi.org/10.4102/jir.v3i1.43.
Exton, J.H. (1972). Gluconeogenesis. Metabolism, 21(10), 945-990.
Guo, Z. (2015). Pyruvate dehydrogenase, Randle cycle, and skeletal muscle insulin resistance. Proceedings of the National Academy of Sciences, 112(22), E2854. https://doi.org/10.1073/pnas.1505398112
Hatting, M., Tavares, C.D., Sharabi, K., Rines, A.K. and Puigserver, P. (2018). Insulin regulation of gluconeogenesis. Annals of the New York Academy of Sciences, 1411(1), 21-35.
Hue, L. and Taegtmeyer, H. (2009). The Randle cycle revisited: a new head for an old hat. American Journal of Physiology-Endocrinology and Metabolism, 297(3), E578-E591.
Kelley, D.E. and Mandarino, L.J. (2000). Fuel selection in human skeletal muscle in insulin resistance: a reexamination. Diabetes, 49(5), 677-683.
Kraus-Friedmann, N. (1984). Hormonal regulation of hepatic gluconeogenesis. Physiological Reviews, 64(1), 170-259.
Li, J., Stillman, J. S., Clore, J. N., & Blackard, W. G. (1993). Skeletal muscle lipids and glycogen mask substrate competition (Randle cycle). Metabolism, 42(4), 451-456. https://doi.org/10.1016/0026-0495(93)90102-T
Lorenz, M. A., El Azzouny, M. A., Kennedy, R. T., & Burant, C. F. (2013). Metabolome response to glucose in the β-cell line INS-1 832/13. Journal of Biological Chemistry, 288(15), 10923-10935. https://doi.org/10.1074/jbc.M112.414961
Marcelino, H., Veyrat-Durebex, C., Summermatter, S., Sarafian, D., Miles-Chan, J., Arsenijevic, D., Zani, F., Montani, J.P., Seydoux, J., Solinas, G. and Rohner-Jeanrenaud, F. (2013). A role for adipose tissue de novo lipogenesis in glucose homeostasis during catch-up growth: a Randle cycle favoring fat storage. Diabetes, 62(2), 362-372.
Melkonian EA, Asuka E, Schury MP. Physiology, Gluconeogenesis. In: StatPearls. StatPearls Publishing, Treasure Island (FL); 2023. PMID: 31082163.
https://europepmc.org/article/nbk/nbk541119
Prasad, A. (2017). Randle Cycle as Applied to Diabetes Mellitus Type 2 ‘Spruce the Basement before Dusting the Super-structure’. International Journal of Biochemistry Research & Review, 18(4), 1-10.
Randle, P., Garland, P., Hales, C., & Newsholme, E. (1963). The glucose fatty-acid cycle: Its role in insulin sensitivity and the metabolic disturbances of diabetes mellitus. The Lancet, 281(7285), 785-789. https://doi.org/10.1016/S0140-6736(63)91500-9
Randle, P.J. (1998). Regulatory interactions between lipids and carbohydrates: the glucose fatty acid cycle after 35 years. Diabetes/metabolism reviews, 14(4), 263-283.
Schulze, T., Morsi, M., Reckers, K., Brüning, D., Seemann, N., Panten, U. and Rustenbeck, I., 2017. Metabolic amplification of insulin secretion is differentially desensitized by depolarization in the absence of exogenous fuels. Metabolism, [online] 67, pp.1–13.
https://doi.org/10.1016/j.metabol.2016.10.008.
Thiébaud, D., DeFronzo, R. A., Jacot, E., Golay, A., Acheson, K., Maeder, E., Jéquier, E., & Felber, J. (1982). Effect of long chain triglyceride infusion on glucose metabolism in man. Metabolism, 31(11), 1128-1136. https://doi.org/10.1016/0026-0495(82)90163-9
Wolfe, B. M., Klein, S., Peters, E. J., Schmidt, B. F., & Wolfe, R. R. (1988). Effect of elevated free fatty acids on glucose oxidation in normal humans. Metabolism, 37(4), 323-329. https://doi.org/10.1016/0026-0495(88)90131-X
Zhang, X., Yang, S., Chen, J., & Su, Z. (2019). Unraveling the regulation of hepatic gluconeogenesis. Frontiers in Endocrinology, 9, 802.
Ceruloplasmin: The Master Antioxidant
Key Takeaways: Ceruloplasmin is a copper-containing enzyme essential for iron metabolism and preventing oxidative stress. It helps transport iron safely, preventing iron overload in tissues…
Adrenal Cocktail: Recipe and Benefits
Key Takeaways The adrenal cocktail supports adrenal health and maintains energy levels. Combines potassium, sodium, and vitamin C for effective adrenal nourishment. Consumed in the…
Diatomaceous Earth: Natural Uses & Benefits
Key Takeaways – Diatomaceous earth is a natural powder made from fossilized algae called diatoms. – It helps cleanse the body of toxins and heavy…
Oxidative Stress: Causes, Effects, Solutions
Key Takeaways Oxidative stress results from an imbalance between free radicals and antioxidants in the body, leading to cellular damage. Chronic oxidative stress contributes to…
Melatonin: Functions and Benefits
Key Takeaways Melatonin helps regulate sleep-wake cycles, signaling the body to rest as it gets dark. It acts as an antioxidant, protecting cells from damage….
Adrenal Fatigue: Symptoms & Prevention
Key Takeaways: Adrenal fatigue is often linked to prolonged stress, leading to tiredness, brain fog, and mood swings. Disruptions in cortisol production can affect energy,…
The EWG Dirty Dozen: What You Need to Know
Key Takeaways The Dirty Dozen list highlights fruits and vegetables with the highest levels of pesticide residues. In 2024, strawberries, spinach, and kale top the…
Fluoride: Risks & Controversies
Key Takeaways Fluoride is widely used in dental products and water supplies, but its safety is debated. Overexposure to fluoride can lead to conditions like…
Are Energy Drinks Dangerous?
Key Takeaways: Caffeine is the most common stimulant in energy drinks. Sugar, though harmful, is widely used in energy drinks. Electrolytes help maintain hydration and…
Autism: Causes, Symptoms, and Management
Key Takeaways Autism Spectrum Disorder (ASD) is a complex neurodevelopmental condition that varies widely in symptoms and severity. Both genetic and environmental factors contribute to…
11 Amazing Tips to Improve Your Sleep Quality
Limit Power NapsModulate Sunlight ExposurePay Attention to CaffeineSchedule BedtimePlan Ahead for DinnertimeMelatonin: Not what you thoughtSleep EnvironmentHot Bath or ShowerEliminate Blue LightSleep StackAdrenal CocktailMagnesium The…
GABA (gamma-aminobutyric acid)
DNA & Longevity: Can You Live to 200?
Key Takeaways: Longevity is shaped by a mix of genetics and lifestyle. Certain genes are linked to longer lifespans. Lifestyle choices can influence how long…
Natural Remedies for Common Ailments: From Headaches to Allergies
Key Takeaways The appeal of natural remedies lies in their holistic approach, fewer side effects, and environmental sustainability. Specific natural remedies can effectively alleviate common…
Sunburn Prevention: Holistic and Natural Approaches
Key Takeaways A poor diet increases the risk of sunburn and skin damage. Short, regular sun exposure reduces the risk of sunburn. Early morning and…
Gout: Symptoms & Natural Treatment
Key Takeaways Gout results from the accumulation of uric acid crystals in the joints, causing severe pain and inflammation. High uric acid levels are often…
Managing Menopause Symptoms – A Guide to Navigate this Life Stage
Exercise RoutineManaging Stress Improving Sleep HabitsSeeking Emotional Support:Adjusting Your DietConsidering Alternative TherapiesFrequently Asked Questions Menopause is a natural stage in a woman’s life marking the…
Do Artificial Sweeteners Cause Weight Gain? The Surprising Truth
Key Takeaways – Artificial sweeteners may disrupt gut microbiome balance, impacting digestion and immune health. – These sweeteners can interfere with natural metabolism, leading to…
Bromate: Its Impact on Your Thyroid & Nervous System
Key Takeaways Bromate is a toxic byproduct from water disinfection, impacting thyroid and nervous system health. It interferes with iodine, leading to thyroid dysfunction and…
How Insulin Regulates Blood Sugar
Key Takeaways Insulin helps regulate blood sugar by moving glucose into cells. Imbalances in insulin levels can cause conditions like diabetes. Insulin resistance can lead…
Superoxide Dismutase: Your Body’s Antioxidant Defender
Key Takeaways SOD protects against oxidative stress by neutralizing free radicals. Copper is necessary for SOD to function. Low SOD activity can lead to aging,…
Does Grounding or Earthing Actually Work?
Elimination Diets: Find the Foods Behind Your Symptoms
Key Takeaways Elimination diets identify food intolerances by removing and reintroducing specific foods. Divided into two phases: elimination and reintroduction. Items like gluten, soy, and…
Why Sunlight is Essential for a Healthy Life
Key Takeaways Sunlight helps the body produce vitamin D, supporting bone health and immune function. Exposure to sunlight can improve mood and reduce symptoms of…
Osteoarthritis Symptoms & Home Remedies
Key Takeaways Lifestyle adjustments and alternative therapies contribute to overall symptom management. Low-impact exercises and physical activity help maintain mobility and reduce pain. Heat and…
Gestational Diabetes Management: Expert Tips for Success
Key Highlights Gestational diabetes, marked by glucose intolerance during pregnancy, requires careful blood sugar control. A healthy pregnancy with gestational diabetes includes regular exercise, a…