Key Takeaways
- Oxidative stress results from an imbalance between free radicals and antioxidants in the body, leading to cellular damage.
- Chronic oxidative stress contributes to diseases such as cancer, cardiovascular conditions, and neurodegenerative disorders.
- Poor diet, smoking, and exposure to environmental pollutants significantly contribute to oxidative stress.
- Managing oxidative stress involves increasing antioxidant intake, adopting a healthy lifestyle, and reducing exposure to toxins.
- Consuming antioxidant-rich foods, regular exercise, and avoiding processed foods effectively combat oxidative stress.
Introduction to Oxidative Stress
Oxidative stress occurs when free radicals outnumber antioxidants in the body. Free radicals are unstable molecules that cause cellular damage, while antioxidants neutralize them.
An imbalance between the two leads to oxidative stress, which plays a role in aging and disease development.
Causes of Oxidative Stress
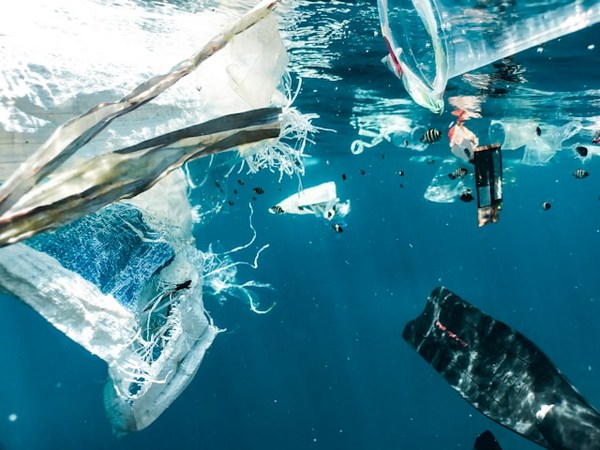
Environmental pollutants, smoking, poor diet, and psychological stress increase free radical production.
Natural biological processes, like metabolism and aging, also contribute to oxidative stress over time.
Iron
While necessary for life, iron can also be a source of harm when present in excess or when it is not properly managed.
This harmful side of iron emerges when it contributes to oxidative stress—a process that can lead to significant cellular damage and a range of chronic health issues.
Iron and Free Radical Production
Iron contributes to oxidative stress through its involvement in the Fenton reaction, a chemical process that produces reactive oxygen species (ROS).
ROS are highly reactive molecules that can damage cellular structures, including DNA, proteins, and lipids.
This damage disrupts normal cellular functions and can trigger a cascade of negative health effects, including inflammation and the development of chronic diseases.
Iron Regulation and Oxidative Stress
Hepcidin is a peptide hormone that plays a central role in regulating iron levels in the body. During times of inflammation, the production of hepcidin increases, leading to reduced iron absorption from the diet and trapping of iron within cells.
This iron retention, while a natural defense mechanism against infections, can lead to an excess buildup of iron in tissues, contributing to oxidative stress and tissue damage.
Copper and Ceruloplasmin
Copper is an essential mineral that, through its involvement with ceruloplasmin, regulates iron metabolism.
Ceruloplasmin is a protein that facilitates the safe transport and storage of iron by oxidizing ferrous iron (Fe2+) to its less reactive ferric form (Fe3+), preventing it from contributing to oxidative stress.
When there is a deficiency in bioavailable copper, ceruloplasmin activity diminishes, leading to iron dysregulation and an increased risk of oxidative stress.
Effects of Oxidative Stress on the Body
Oxidative stress accelerates aging and damages cells, proteins, and DNA. It’s linked to cardiovascular diseases, cancer, neurodegenerative diseases like Alzheimer’s, and diabetes.
Health Complications
Autoimmune Diseases:
The oxidative stress caused by iron can damage tissues and organs, triggering abnormal immune responses where the body attacks its own cells.
Cardiovascular Diseases:
Excess iron can promote the formation of arterial plaques, leading to conditions like atherosclerosis, heart attacks, and strokes.
Neurodegenerative Disorders:
The brain is particularly vulnerable to oxidative stress due to its high oxygen consumption and abundance of easily oxidized fats.
Iron accumulation in the brain has been associated with neurodegenerative diseases such as Alzheimer’s and Parkinson’s disease, where oxidative stress contributes to the progressive loss of neuronal function.
Symptoms and Indicators of Oxidative Stress
Common symptoms include fatigue, memory loss, and premature aging. Biomarkers like 8-hydroxydeoxyguanosine (8-OHdG) and malondialdehyde (MDA) can measure oxidative stress levels.
Strategies to Mitigate Oxidative Stress
Increasing antioxidant intake through diet, engaging in regular exercise, and reducing exposure to toxins are key strategies.
Antioxidant-rich foods include organ meats, fatty fish, berries, leafy greens, and nuts.
Regular physical activity boosts antioxidant defenses, while stress management techniques like meditation help lower oxidative stress levels.
Role of Antioxidants in Combating Oxidative Stress
Antioxidants neutralize free radicals. Key antioxidants include vitamins C and E, glutathione, and selenium.
These can be found in liver, eggs, fish, fruits, vegetables, and nuts.
Our newest paper is now published!
— Isabella Cooper (@I_mitochondria) July 12, 2024
Ketosis Suppression and Ageing (KetoSAge) Part 2: The Effect of Suppressing Ketosis on Biomarkers Associated with Ageing, HOMA-IR, Leptin, Osteocalcin, and GLP-1, in Healthy Females
Metabolic dysfunctions are among the best documented… pic.twitter.com/wjSrhvC737
Medical and Supplement Interventions
Antioxidant supplements, such as whole food vitamin C complex, vitamin E, and coenzyme Q10, can help manage oxidative stress.
Conclusion
Maintaining a balance between free radicals and antioxidants is essential for health. Simple lifestyle and dietary changes can help mitigate oxidative stress and its associated risks.Consulting with a healthcare provider ensures the best approach to supplementation.
FAQ
What is oxidative stress and why is it harmful?
Oxidative stress is an imbalance between free radicals and antioxidants, leading to cellular damage and various diseases.
What are common causes of oxidative stress?
Environmental pollutants, poor diet, smoking, excessive alcohol consumption, and psychological stress are common causes.
How can I reduce oxidative stress in my body?
Adopting a diet rich in antioxidants, regular exercise, reducing exposure to toxins, and managing stress can help reduce oxidative stress.
What foods are high in antioxidants?
Animal foods like organ meats and fatty fish, along with fruits, vegetables, and nuts, are high in antioxidants.
Can supplements help with oxidative stress?
Antioxidant supplements may help, but it’s best to consult a healthcare provider for personalized advice.
Research
Batey RG, Lai Chung Fong P, Shamir S, Sherlock S. A non-transferrin-bound serum iron in idiopathic hemochromatosis. Dig Dis Sci. 1980 May;25(5):340-6. doi: 10.1007/BF01308057. PMID: 7371472.
Bo, S., Durazzo, M., Gambino, R., Berutti, C., Milanesio, N., Caropreso, A., Gentile, L., Cassader, M., Cavallo-Perin, P. and Pagano, G., 2008. Associations of Dietary and Serum Copper with Inflammation, Oxidative Stress, and Metabolic Variables in Adults ,. The Journal of Nutrition, [online] 138(2), pp.305–310. https://doi.org/10.1093/jn/138.2.305.
Boddaert, N., Le Quan Sang, K. H., Rötig, A., Leroy-Willig, A., Gallet, S., Brunelle, F., Sidi, D., Thalabard, J., Munnich, A., & Cabantchik, Z. I. (2007). Selective iron chelation in Friedreich ataxia: Biologic and clinical implications. Blood, 110(1), 401-408. https://doi.org/10.1182/blood-2006-12-065433
DiNicolantonio, J.J., Mangan, D. and O’Keefe, J.H., 2018. The fructose–copper connection: Added sugars induce fatty liver and insulin resistance via copper deficiency. Journal of Metabolic Health, [online] 3(1).
https://doi.org/10.4102/jir.v3i1.43.
Fillebeen, C., Descamps, L., Dehouck, M.-P., Fenart, L., Benaïssa, M., Spik, G., Cecchelli, R. and Pierce, A., 1999. Receptor-mediated Transcytosis of Lactoferrin through the Blood-Brain Barrier. Journal of Biological Chemistry, [online] 274(11), pp.7011–7017. https://doi.org/10.1074/jbc.274.11.7011.
Gaetke, L., 2003. Copper toxicity, oxidative stress, and antioxidant nutrients. Toxicology, [online] 189(1–2), pp.147–163. https://doi.org/10.1016/s0300-483x(03)00159-8.
Galaris, D., Barbouti, A. and Pantopoulos, K., 2019. Iron homeostasis and oxidative stress: An intimate relationship. Biochimica et Biophysica Acta (BBA) - Molecular Cell Research, [online] 1866(12), p.118535. https://doi.org/10.1016/j.bbamcr.2019.118535.
Greenberg, G.R. and Wintrobe, M.M., 1946. A LABILE IRON POOL. Journal of Biological Chemistry, [online] 165(1), pp.397–398. https://doi.org/10.1016/s0021-9258(17)41250-6.
Gutteridge, J.M.C. and Halliwell, B., 2018. Mini-Review: Oxidative stress, redox stress or redox success? Biochemical and Biophysical Research Communications, [online] 502(2), pp.183–186.
https://doi.org/10.1016/j.bbrc.2018.05.045.
Hentze, M.W., Muckenthaler, M.U., Galy, B. and Camaschella, C., 2010. Two to Tango: Regulation of Mammalian Iron Metabolism. Cell, [online] 142(1), pp.24–38. https://doi.org/10.1016/j.cell.2010.06.028.
Ke, Y. and Qian, Z.M., 2007. Brain iron metabolism: Neurobiology and neurochemistry. Progress in Neurobiology, [online] 83(3), pp.149–173. https://doi.org/10.1016/j.pneurobio.2007.07.009.
Kenkhuis, B., Bush, A.I. and Ayton, S., 2023. How iron can drive neurodegeneration. Trends in Neurosciences, [online] 46(5), pp.333–335.
https://doi.org/10.1016/j.tins.2023.02.003.
Kruszewski, M., 2003. Labile iron pool: the main determinant of cellular response to oxidative stress. Mutation Research/Fundamental and Molecular Mechanisms of Mutagenesis, [online] 531(1–2), pp.81–92.
https://doi.org/10.1016/j.mrfmmm.2003.08.004.
Milanino, R., Conforti, A., Franco, L., Marrella, M. and Velo, G., 1985. Review: Copper and inflammation — a possible rationale for the pharmalogical manipulation of inflammatory discorders. Agents and Actions, [online] 16(6), pp.504–513. https://doi.org/10.1007/bf01983655.
Mills, E., Dong, X., Wang, F. and Xu, H., 2009. Mechanisms of Brain Iron Transport: Insight into Neurodegeneration and CNS Disorders. Future Medicinal Chemistry, [online] 2(1), pp.51–64. https://doi.org/10.4155/fmc.09.140.
Moos T., Morgan EH. Transferrin and transferrin receptor function in brain barrier systems. Cell Mol Neurobiol. 2000 Feb;20(1):77-95. doi: 10.1023/a:1006948027674. PMID: 10690503.
Moos, T., Nielsen, T.R., Skjørringe, T. and Morgan, E.H., 2007. Iron trafficking inside the brain. Journal of Neurochemistry, [online] 103(5), pp.1730–1740. https://doi.org/10.1111/j.1471-4159.2007.04976.x.
Muckenthaler MU, Galy B, Hentze MW. Systemic iron homeostasis and the iron-responsive element/iron-regulatory protein (IRE/IRP) regulatory network. Annu Rev Nutr. 2008;28:197-213. doi: 10.1146/annurev.nutr.28.061807.155521. PMID: 18489257.
Sorenson, J.R.J., 1989. 6 Copper Complexes Offer a Physiological Approach to Treatment of Chronic Diseases. Progress in Medicinal Chemistry, [online] pp.437–568. https://doi.org/10.1016/s0079-6468(08)70246-7.
Uriu-Adams, J.Y. and Keen, C.L., 2005. Copper, oxidative stress, and human health. Molecular Aspects of Medicine, [online] 26(4–5), pp.268–298. https://doi.org/10.1016/j.mam.2005.07.015.
Wang, J. and Pantopoulos, K., 2011. Regulation of cellular iron metabolism. Biochemical Journal, [online] 434(3), pp.365–381. https://doi.org/10.1042/bj20101825.
Wallander, M.L., Leibold, E.A. and Eisenstein, R.S., 2006. Molecular control of vertebrate iron homeostasis by iron regulatory proteins. Biochimica et Biophysica Acta (BBA) - Molecular Cell Research, [online] 1763(7), pp.668–689. https://doi.org/10.1016/j.bbamcr.2006.05.004.
Ward, R.J., Zucca, F.A., Duyn, J.H., Crichton, R.R. and Zecca, L., 2014. The role of iron in brain ageing and neurodegenerative disorders. The Lancet Neurology, [online] 13(10), pp.1045–1060.
https://doi.org/10.1016/s1474-4422(14)70117-6.
Is Eating Sugar Really That Bad For Your Health?
Should You Really Be Concerned? In short, YES! Thank you, that’s all folks, and do have a good evening. Seriously though, extensive research has established…
How Cod Liver Oil Can Transform Your Health and Wellness
Cod liver oil has been used for centuries as a natural remedy for various health conditions. Packed with essential nutrients and fatty acids, cod liver…
Spirulina: Health Benefits and Uses
Key Takeaways Spirulina boosts immune function with its high nutrient content and antioxidant properties. Rich in proteins and essential vitamins, enhances overall nutrition. Helps reduce…
13 Most Dangerous Foods Revealed
Key Highlights Fugu, or pufferfish, is one of the most poisonous foods in the world, with its organs containing a neurotoxin that can paralyze motor…
Trimethylglycine TMG: Betaine Anhydrous Explained
Key Takeaways Betaine Anhydrous (TMG) is a compound found naturally in various foods and offers several health benefits. TMG supports liver health by reducing fatty…
Medium Chain Triglycerides (MCTs): Uncovering 5 Health Benefits
This potent, natural source of energy has gained considerable attention in recent years for its impressive array of benefits. MCT oil is a versatile addition…
How Collagen Supports Healthy Skin, Joints, and More
Key Takeaways Collagen is the most abundant protein in the body, supporting the structure of skin, bones, and connective tissues. It helps maintain skin elasticity,…
Creatine Myths Debunked: Separating Fact from Fiction
Key Takeaways Common myths about creatine, such as it causing kidney damage, weight gain, and being a steroid, are widespread but unsupported by scientific evidence….
Potassium: Benefits & Sources
Key Takeaways Potassium is essential for regulating fluid balance, nerve signals, and muscle function. It supports heart health and helps maintain proper blood pressure. Adequate…
Copper: Little-Known Health Benefits
Key Takeaways Copper is an essential trace mineral with benefits, including ceruloplasmin production, energy production and antioxidant properties. Copper is critical for brain health by…
Magnesium: Better Sleep, Stress Relief and More
Tallow: Benefits, Uses, and Nutrition
Key Takeaways: Tallow is a nutrient-rich animal fat with many practical uses. It contains valuable vitamins such as A, D, E, and K. Tallow is…
Natural Treatment for Irritable Bowel Syndrome (IBS): Effective Remedies Explored
Understanding IBSSymptoms of IBSRole of Diet in IBSNatural Remedies for IBSSupplements for IBSRole of Probiotics in IBSFrequently Asked Questions Understanding IBS Irritable Bowel Syndrome (IBS)…
Healthy Fat: is Butter Better?
Key Takeaways Saturated fats, like those found in butter, may not be as harmful as once thought and can be part of a healthy diet….
What You Need to Know About Salt and Your Health
Table of ContentsThe Health Benefits of Unrefined Sea SaltElectrolyte BalanceMineral ContentImproved HydrationBoosted Energy LevelsImmune SupportImproved DigestionBalanced pH LevelsReduced Water RetentionHeart Health SupportStronger Bones and TeethEnhanced…
Red Palm Oil: Unveiling The Potent Health Benefits
Struggling to find the right oil for your health and kitchen? Red palm oil is packed with nutrients that might just be what you need….
Boron: Benefits of a Lesser-Known Mineral
Key Takeaways Boron is a trace mineral with significant health benefits. It supports brain function, bone health, and hormonal balance. Understanding boron’s role can improve…
6 Best Natural Ways to Manage Your Blood Sugar: A Quick & Easy Guide
1. Intermittent fasting2. Exercise3. Dietary fiber4. Sleep5. Weight loss6. SupplementationBioclinic NaturalsPGX BiotiquestSugar Shift Every time you eat it, it’s plotting something sinister. Sugar isn’t as…
TUDCA Benefits for Health
Key Takeaways TUDCA promotes liver health, aiding cell protection and repair. Enhances digestion by improving bile flow and supporting gut health. May protect brain health…
Allulose: The Best Sugar Alternative
Key Takeaways Allulose is a low-calorie sweetener found naturally in some fruits. It does not raise blood sugar levels, making it suitable for diabetics. Allulose…
11 Electrifying Health Benefits of Trace Minerals
What are Trace Minerals?The Major Roles of Trace MineralsSources of Trace MineralsDeficiencies in Trace MineralsThe Impact of Trace Minerals on Specific Health ConditionsFrequently Asked Questions…
Whole Food Vitamin C Complex: Expert Tips for Health
Key Highlights Whole food vitamin C complex is essential for a strong immune system and overall health. Unlike synthetic ascorbic acid, whole food vitamin C…
8 Key Signs of Nutrient Deficiency
Key Takeaways Magnesium: A multitasker that aids in over 300 biochemical reactions in the body. Copper: Supports neurological function, cardiovascular and immune system health, iron…
Calcium Supplements: What You Need to Know
Key Takeaways Calcium supplements have been linked to heart disease and kidney stones. Excess calcium from supplements can lead to imbalances and health issues. Natural…
Actual Superfoods: Real Foods You Should Be Eating
Key Takeaways Superfoods are nutrient-dense foods, offering essential vitamins, minerals, and fats. Prioritize high-quality sources for optimal nutrition. They support overall health, boost energy, and…
Eggs: A Comprehensive Guide
Key Highlights Eggs are a nutritional powerhouse, containing all the essential vitamins and minerals needed for overall health. Vital role in a balanced diet, providing…
Benefits of Nutritional Yeast
Key Takeaways Nutritional yeast is a rich source of vitamins and minerals. It supports immune function and promotes skin health. Its cheesy flavor makes it…
Cholesterol Misconceptions: Separating Fact from Fiction
Key Takeaways: High inflammation and blood pressure are major risk factors for heart disease. Cholesterol is vital for hormone production, cell membrane structure, and digestion,…
Do This! The Ultimate Guide to Fasting Safely and Effectively
In our increasingly busy lives, finding time to take care of our bodies can often take a backseat. One method that has gained attention recently…
Vitamin E Complex
Key Takeaways Vitamin E is a powerful antioxidant that protects cells from oxidative damage, reducing the risk of chronic diseases. The vitamin E complex includes…
Increase GLP-1 Agonists Naturally
Key Takeaways: GLP-1 agonists regulate appetite, insulin production, and blood sugar levels. Regular exercise and quality sleep maintain optimal GLP-1 levels. High-protein, low-carb diets effectively…
Iron Overload: Symptoms & Prevention Tips
Key Takeaways: Iron overload happens when the body absorbs excessive iron, which can damage organs. Common symptoms include fatigue, joint pain, and skin changes. Early…
Benefits of Sea Moss Explained
Key Takeaways Rich in Nutrients: Sea moss is packed with essential vitamins, minerals, and antioxidants, supporting overall health and wellness. Supports Immune Function: Its high…
L-Glutamine and Gut Health: Benefits and Side Effects
Key Takeaways L-Glutamine is essential for gut health. Benefits include improved digestion and reduced inflammation. Potential side effects are rare but can occur in high…
Carnivore Diet: Benefits, Risks, Food List & More
Key Takeaways The carnivore diet is a keto diet that only allows for animal-based foods, and has potential health benefits. Tips for success include hydrating,…
Keto Diet 101: A Complete Beginner’s Guide
Key Highlights The ketogenic diet is a low-carb, high-fat diet that can lead to weight loss and has many health benefits. By reducing carbohydrate intake…
ALA vs. DHA & EPA Omega-3: Why Source Matters
Key Takeaways ALA (Alpha-Linolenic Acid) is found in flaxseeds, chia seeds, and walnuts, but converts poorly to DHA and EPA. DHA and EPA are critical…
Silica: for Healthier Skin, Hair, and Nails
Key Takeaways: Silica supports strong and healthy skin, hair, and nails. It promotes bone health by boosting collagen production. Silica helps improve joint flexibility and…
The Impact of Ultra-Processed Foods on Your Wellbeing
Every bite we take is a step toward either wellness or illness. In our fast-paced world, ultra-processed foods have become a staple, silently shaping our…
Liver: 5 Surprising Benefits Backed by Science
Hold on! Don’t run away! You need to read this. Liver is a highly nutritious organ meat that is often overlooked in modern diets. Packed…
Berberine Has 11 More Incredible Benefits Than You Thought
Berberine is a compound found in several plants that has been used for centuries in traditional Chinese medicine and Ayurveda. It has recently gained popularity…
Zinc Supplements: Risks and Dangers
Key Takeaways Zinc supports immunity, wound healing, and cell growth. High zinc supplement doses can cause health problems. Always consult a healthcare provider before taking…
L-Carnitine: Benefits, Dosage, and Side Effects
Key Takeaways L-Carnitine supports fat metabolism and energy production. Benefits include enhanced exercise performance and improved heart health. Proper dosing minimizes potential side effects. Understanding…
Bee Pollen: Nature’s Secret Superfood
Key Takeaways Bee pollen is packed with essential nutrients and offers numerous health benefits. It supports immune function, boosts energy, and promotes overall well-being. Adding…
5-HTP: Natural Ways to Boost Serotonin and Improve Mood
Key Takeaways: 5-HTP is a natural compound that helps boost serotonin levels in the brain. It can support mood regulation, sleep improvement, and stress reduction….
5 Major Benefits of Omega-3 Fatty Acids
Key Takeaways Omega-3 fatty acids support heart health by reducing triglycerides and lowering blood pressure. They play an important role in brain function and development,…
CoQ10: What Is It and Why Is It Important?
Key Takeaways CoQ10 (Coenzyme Q10) is an antioxidant produced by the body, essential for energy production in cells. Levels of CoQ10 naturally decrease with age…
Conjugated Linoleic Acid (CLA): Benefits & Sources
Key Takeaways CLA is a type of fatty acid found primarily in animal products like beef and dairy. Known for potential benefits such as weight…
Postbiotics: What They Are and Why They Are Important
Key Takeaways Postbiotics 101: They’re beneficial by-products from probiotics that consume prebiotics Boosts Immunity: Postbiotics sharpen your immune system, helping fight off pathogens and reducing…
Vitamin A (Retinol): Essential Nutrient for Health
Key Takeaways: Natural Vitamin A, also known as Retinol, is crucial for vision, immune function, and skin health. Retinol is essential for healthy vision, particularly…
Taurine: The Mighty Amino Acid for Optimal Health
Key Takeaways Taurine supports heart health, regulates blood pressure, and reduces oxidative stress. Essential for muscle function, brain health, and cognitive function. Aids in insulin…
Protein: You probably need more
Key Takeaways Protein is needed for building and repairing body tissues. It supports muscle growth, immune function, and hormone production. Bioavailable sources of protein include…